Fred Magdoff: Creating an ecological civilisation
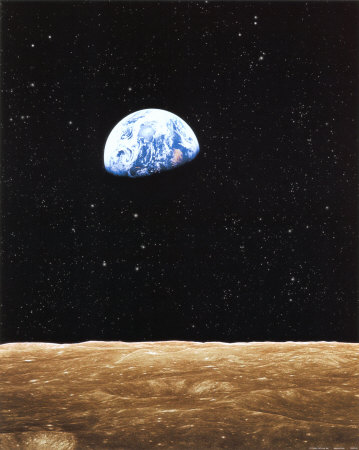
By Fred Magdoff
“It is inconceivable that capitalism itself will lead directly to an ecological civilization that provides the basic needs for all people. However, building an ecological civilization that is socially just will not automatically happen in post-capitalist societies. It will occur only through the concerted action and constant vigilance of an engaged population.''
January 2011 – Monthly Review – Given the overwhelming harm being done to the world’s environment and to its people, it is essential today to consider how we might organize a truly ecological civilization—one that exists in harmony with natural systems—instead of trying to overwhelm and dominate nature. This is not just an ethical issue; it is essential for our survival as a species and the survival of many other species that we reverse the degradation of the earth’s life support systems that once provided dependable climate, clean air, clean water (fresh and ocean), bountiful oceans, and healthy and productive soils.
There are numerous ways to approach and think about the enormous harm that has been done to the environment. I will discuss the following: (1) the critical characteristics that underlie strong ecosystems; (2) why societies are not adequately implementing ecological approaches; and (3) how we might use characteristics of strong natural ecosystems as a framework to consider a future ecological civilization.
I. Ecological principles: learning from nature
The study of ecology developed as scientists began to understand how natural systems functioned. Scientists quickly realized that they needed to think and study in a multidisciplinary fashion—there was no way to comprehend the full complexity of such systems by focusing on one particular discipline or sub-discipline. In fact, even after intense study, it is usually impossible to understand fully a highly complex system—with interactions and positive and negative feedback loops—to the extent that you can accurately predict all the results that will occur when you intervene in some way. Frederick Engels described this phenomenon well, over a century ago in 1876:
Let us not … flatter ourselves overmuch on account of our human victories over nature. For each such victory nature takes its revenge on us. Each victory, it is true, in the first place brings about the results we expected, but in the second and third places it has quite different, unforeseen effects which only too often cancel out the first. The people who, in Mesopotamia, Greece, Asia Minor, and elsewhere, destroyed the forests to obtain cultivable land, never dreamed that by removing along with the forests the collecting centres and reservoirs of moisture they were laying the basis for the present forlorn state of those countries. When the Italians of the Alps used up the pine forests on the southern slopes, so carefully cherished on the northern slopes, they had no inkling that by doing so they were cutting at the roots of the dairy industry in their region; they had still less inkling that they were thereby depriving their mountain springs of water for the greater part of the year, and making it possible for them to pour still more furious torrents on the plains during the rainy seasons ...Thus at every step we are reminded that we by no means rule over nature like a conqueror over a foreign people, like someone standing outside nature—but that we, with flesh, blood and brain, belong to nature, and exist in its midst, and that all our mastery of it consists in the fact that we have the advantage over all other creatures of being able to learn its laws and apply them correctly.[1]
Learning to “know and correctly apply” the “laws” of nature has progressed greatly since Engels’s time. Although we must always proceed with caution when working with complex ecosystems (as Engels warned, there may be unforeseen consequences), much has been learned about how natural systems operate, about the importance of the interactions of organisms among themselves and with their physical/chemical/climatic environment. There are fragile natural ecosystems that are easily disturbed, and may become degraded as a result of slight disturbance. However, many natural ecosystems are strong, able to resist significant perturbation and/or quickly return to normal functioning following a disturbance. Natural disturbances of an ecosystem may be sudden—a wildfire started by a lightening strike, huge winds generated by hurricanes, floods, etc.—or gradual, as with changes in long-term precipitation trends. More resilient systems are better able to adapt to long-term gradual changes as well as sudden ones.
Metabolism and metabolic connections
The term metabolism is usually used in reference to the work done inside an organism or a cell as it goes about its normal operations: the building up of new organic chemicals and the breaking down of others, the recovering of energy from some compounds, and the use of energy to do work. But a critical part of the metabolism of a cell or large organism is the exchange of materials with the environment and other organisms: obtaining energy-rich organic molecules and individual elements necessary to make all the stuff of life, including oxygen, carbon dioxide, nutrients (such as nitrogen, phosphorus, potassium, and calcium), and water. Without access to these resources outside itself, an organism would run out of energy and die. Plants and animals, as well as fungi and most bacteria, need to be able to obtain oxygen from the atmosphere or water in order to live. In addition, all organisms must rid themselves of waste products such as carbon dioxide that can be toxic if allowed to accumulate inside the organism. Thus, metabolism involves not only processes internal to the organism but also a continual exchange of materials between an organism and its immediate environment—the soil, air, water, and other organisms. (See Figure 1.)
Almost all organisms use the energy derived from sunlight—either producing it themselves by photosynthesis or feeding on plant material or organisms that have themselves fed on plant material. However, organisms, from the “simplest” bacteria to mammals, interact with one another and with the chemical and physical aspects of their local environment. The waste of one cell—or the whole organism itself—becomes food for another. And many organisms contain other organisms, either inside or on their surfaces, most living in a mutually beneficial, symbiotic association. The huge number of bacteria inside the human gut (as there are inside those of other animals) plays an important part in our metabolism and assists in the normal functioning of the body.
Soil is not just a medium for supporting plants so they can grow upright. It is also composed of minerals, gases (atmosphere), water, decomposing organic material, and literally millions of organisms such as fungi, bacteria, nematodes, earthworms, and so on, all continually interacting and providing resources to one another. There is a strong metabolic interaction between plants and soil. Plants, as they grow, provide food for many soil organisms, as material produced by photosynthesis in green tissue is translocated to the roots and exuded. (See Figure 1, which outlines some of the main metabolic connections in a natural ecosystem in which humans are part.)
Many of the microorganisms close to roots provide organic chemicals that support healthy plant growth. All plants also take up nutrients such as potassium, phosphorus, magnesium, calcium, and nitrogen. Some plants, such as legumes, which function symbiotically with bacteria inside the plants, supply energy-rich products of photosynthesis to the bacteria, which in turn supply the plant with available forms of nitrogen. When plants or plant roots die, they become food for fungi and bacteria that then become energy sources for organisms such as nematodes that then become food for other organisms further up a complex metabolic foodweb of life. (A foodweb is the term now used in place of food chain—because organisms are connected in complex, branching ways and not in an orderly, ladder-like chain.) Although plants make their own energy-rich compounds using the sun’s energy to power photosynthesis, the essential inorganic compounds that plants take up from the soil also participate in the foodweb. As organisms feed on dead plant material, they use energy contained in the residue for their life processes and, at the same time, convert nutrients in their food into forms that can be used by plants in the future.
Plants living in the same ecosystem are frequently connected to one another metabolically. The release of available nitrogen from a legume can, for example, be taken up by a neighboring grass plant. The threads of fungi (hyphae) that help plants take up water and nutrients frequently connect one plant to another. In addition, some plants provide resources and refuge to insects that attack insects that feed on other plants.
Plants and soils are important participants in the hydrologic cycle. Plants take up water from soil and use some for growth, while most water evaporates from leaves, returning to the atmosphere to fall back to Earth again as rainfall. Precipitation may filter into the soil and be stored there for plants to use, or it may percolate down to recharge the water table. If the soil is open and porous and has a cover of organic mulch, a high percentage of rainfall can infiltrate. Conversely, if soils are compact and have no surface organic mulch and few plants, rainfall tends to run off the land, eroding soil as it flows.
There is also an intimate and important metabolic connection between plants and higher animals, as animals feed on plants (or other animals that fed on plants), using nutrients from the soil and solar energy that have been stored in plants. In the living and reproducing processes of animals, energy is used to do work, and nutrients are returned to the soil as waste materials. There are strong animal-to-animal metabolic relationships, as carnivores, omnivores (humans included), parasitoids (insects that parasitize other insects), etc. go about their normal lives.
Humans create forms of metabolic interaction with the earth in many ways—agricultural systems, fishing, mining, production and use of industrial commodities, production and utilization of transportation systems, heating homes and cooking, disposing of solid waste (garbage), changing the landscape to accommodate road construction, etc. However, in this section, we include only human metabolic relationships with the earth as they are expressed through the consumption of food and excretion of bodily waste. This allows us to focus on what can be learned from natural systems.
Strong natural ecosystems
The continuity of the natural soil-plant-animal metabolic connections is critical to maintaining strong ecosystems. And within the soil, the metabolic interactions of the great diversity of organisms help provide the nutrients and stimulating compounds that are essential for growing healthy plants as well as enhancing infiltration of rainfall and storage capacity for water for later use by plants.
A number of characteristics can be thought of as pillars supporting strong natural ecosystems. These have been described as follows:
- Diversity. A great biological diversity, both aboveground and in the soil, characterizes many strong natural ecosystems in temperate and tropical regions. The metabolic connection between so many organisms provides biological synergies, nutrient availability to plants, checks on disease or insect outbreaks, etc. For example, competition for resources and specific antagonisms (such as antibiotic production by some soil bacteria) among the multitude of soil organisms usually keep soil borne plant diseases from severely damaging a natural grassland or forest. And a great diversity in species aboveground (plants and animals) and in the soil, some with overlapping niches or functions, means that if one is harmed by a disease or insect, there are frequently others that promote the continuity of ecosystem functioning.
- Efficient natural cycles through closely linked metabolic relationships. Efficiency of natural systems’ natural cycles is arrived at through close biological interactions and associations and biological synergies. Tight energy and nutrient flows—with little wasted or “leaking out”—are characteristic of strong natural systems. Because of the wide variety and great populations of organisms and their many natural niches in soils and on the soil surface and the presence of significant quantities of stored solar energy in crop and manure residues, energy flows efficiently, as organisms consume organic residues and one another. Natural ecosystems with healthy soils and diverse plant species also tend to be efficient in capturing and using rainfall and in mobilizing and cycling nutrients. This efficiency in use of nutrients, energy, and water helps to keep the ecosystem from “running down” through the excessive loss of energy and nutrients (and topsoil) and, at the same time, helps maintain the quality of the groundwater and surface waters. Precipitation tends to enter the porous soil, rather than run off, providing water to plants and recharging ground water, slowly releasing water to springs, streams, and rivers.
- Self-sufficiency. A consequence of diversity and efficient natural cycles is that natural terrestrial ecosystems are self-sufficient—requiring only inputs of sunlight and rainfall. Natural ecosystems run on current and recently past solar energy (stored as soil organic matter). The sun’s energy, captured by green plants, is then used by many organisms, as fungi and bacteria decompose organic residues and are then fed upon by other organisms, which are themselves fed upon by others, higher up the foodweb.
Cycling of nutrients from soil to plant to animal and back to soil is essential for an ecosystem to be stable and resilient. There are low levels of nutrients, such as nitrates, that come along with rain and are deposited as dust settles. Other nutrients then become available as soil minerals dissolve. But, to a great extent, nutrients cycle and energy flows efficiently within the system. As organisms decompose dead plants and animals, nutrients are recycled at the same time that energy stored in the food source is utilized. The essential nutrient, nitrogen, needed for making proteins, is provided by a number of species of bacteria (free living as well as those living symbiotically on or in plant roots) that are able to convert nitrogen gas in the atmosphere into amino acids, directly usable by plants, and to release mineral forms of nitrogen that plants can also use.
- Self-regulation. With plants and animals filling the numerous niches aboveground, many species are able to exist side-by-side, for instance, in grasslands. But they metabolically interact with one another and are intimately connected. Because of the great diversity of organisms present, outbreaks (or huge population increases) of diseases or insects that severely damage plants or animals are uncommon. For example, a particular beetle that feeds on plants may encounter numerous other species that keep its population from getting too high—“a variety of predatory bugs and beetles in the foliage of the plants, and pathogenic nematodes and fungi that inhabit the soil attack pupating adults below ground.”[2]
Animals have many systems to defend themselves when under attack by other organisms, including, in mammals, their own immune systems. But plants also have a number of defense mechanisms—some stimulated or induced by soil organisms—that help protect them from attack by fungi and bacteria. There are also organisms that prey on (or lay their eggs in) insects that feed on plants. Amazingly, plants being eaten by plant-feeders (herbivores) send out chemical signals that recruit the specific organism to attack the particular plant-feeders.[3]
- Resiliency through self-renewal. Disturbances occur in all ecosystems, natural or not. The stronger ecosystems are more resistant to disturbances and are able to bounce back quicker.[4] Such resiliency is the “bottom line” characteristic of strong and stable ecosystems, with other characteristics contributing to the ability for self-renewal. Seeds stored in the soil, which germinate after a fire are an example of the self-renewal of plants.
Are there lessons to learn from bees?
The natural world contains many interesting examples that may have some analogies in human activity. For example, when beehives grow too large, bees swarm to a temporary location. Scouts go out in different directions and then report back by performing a dance that provides information about the location scouted and its conditions. Other bees congregate around the original scout they think has selected the best site. When enough bees agree on one of the locations, the entire swarm then goes off to build a hive there.[5]
When a large enough number of bees agree about a site, they end up selecting the best one. There is an analogous situation with humans. People in groups, provided with the necessary information, tend to make better decisions than individuals making decisions for the group. A diversity of people (backgrounds, skills, outlooks) allows a group to evaluate issues better. Because they are, as a group, involved in the decision-making process, they feel more ownership of the decision and a desire to help implement it.
II. Why are societies not applying ecological knowledge?
With every day that passes we are acquiring a better understanding of these laws and getting to perceive both the more immediate and the more remote consequences of our interference with the traditional course of nature. In particular, after the mighty advances of natural science in the present century, we are more than ever in a position to realise, and hence to control, also the more remote natural consequences at least of our day-to-day productive activities. But the more this progresses the more will men not only feel, but also know their oneness with nature, and the more impossible will become the senseless and unnatural idea of a contrast between mind and matter, man and nature, soul and body.—Frederick Engels, 1876[6]
Although the science of ecology was born in the nineteenth century, it developed only gradually over the years. During this process, our understanding about the functioning of natural systems and the interaction of organisms with the environment has deepened. How can we reconcile this growing knowledge with the accelerating pace of environmental destruction? The answer is that nearly the entire world is now part of a global capitalist system, which is, at its heart, an anti-environmental economic/social system. Having accumulation of capital without end as its motivating force and only goal, capitalism creates environmental havoc locally, regionally, and globally.
As Engels explained:
As individual capitalists are engaged in production and exchange for the sake of the immediate profit, only the nearest, most immediate results must first be taken into account. As long as an individual manufacturer or merchant sells a manufactured or purchased commodity with the usual coveted profit, he is satisfied, and does not concern himself with what afterwards becomes of the commodity and its purchasers. The same thing applies to the natural effects of the same actions. What cared the Spanish planters in Cuba, who burned down forests on the slopes of the mountains and obtained from the ashes sufficient fertiliser for one generation of very highly profitable coffee trees—what cared they that the heavy tropical rainfall afterwards washed away the unprotected upper stratum of the soil, leaving behind only bare rock![7]
These words are, if anything, more critical today than when Engels wrote them. The accumulation of capital, the driving and motivating force of capitalism, leads naturally to many consequences that harm the environment. The system proceeds assuming—contrary to all evidence—unlimited resources (including cheap energy) and unlimited natural “sinks” for wastes generated.
Human activity breaks or greatly alters the metabolic connections discussed above and tends to impoverish and weaken the ecosystem, making it function less effectively and with less resilience. Although human-induced metabolic disturbances and rifts occurred in the precapitalist era—for example, the cutting down of forests in the Mediterranean region thousands of years ago led to accelerated runoff of rainfall, soil erosion, and the drying of springs in the dry season—the logic of capitalism and the technology it developed, along with the increased number of people on Earth, led to much greater and more intensive disruptions in the natural cycling of matter, affecting not only local and regional ecologies, but global ones as well.
Recognition of problems that arise as metabolic rifts occur commonly brings about interventions to counteract the effects, or attempts to shift the problems around. But the result more often than not, as Engels pointed out, is the emergence of unanticipated secondary and tertiary effects, many of which are extremely detrimental. The effects of both the ecological rifts and their attempted remedies are local, regional, and global. Hence, there are numerous examples of severe degradation, if not the actual breaking of soil-plant-animal-atmospheric metabolic relationships. (See Figure 2 for rifts during development of cities and capitalist agriculture; Figure 3 for consequences of interventions to try to deal with rifts; and Table 1 on the entire set of disturbances and their consequences.)
Rifts in nutrient cycles (see [a] in Figures 2 and 3)
A number of nineteenth-century observers were concerned with an aspect of capitalism that is still a problem today, though in an increasingly egregious form—the breaking of the cycle of nutrients from soil to plants and farm animals to humans and back to soil, with all its ramifications. For example, Marx wrote in Volume I of Capital:
Capitalist production collects the population together in great centres, and causes the urban population to achieve an ever-growing preponderance….[I]t disturbs the metabolic interaction between man and the earth, i.e., prevents the return to the soil of its constituent elements consumed by man in the form of food and clothing; hence it hinders the operation of the eternal natural condition for the lasting fertility of the soil.[8]
Until the discovery of phosphate deposits and the practice of making the phosphorus in them more available to plants, one of the sources of phosphorus for European agriculture became the bones of former soldiers—which were harvested from the Napoleonic battlefields and burial grounds. The quest for nutrients to replenish soils in the later part of the nineteenth century led to “guano imperialism,” as countries competed to capture islands rich in this natural fertilizer.[9] Peru had the world’s richest guano deposits and was the center of the international guano trade.
Figure 1. Soil-plant-animal-atmosphere metabolic interactions (click image to enlarge).
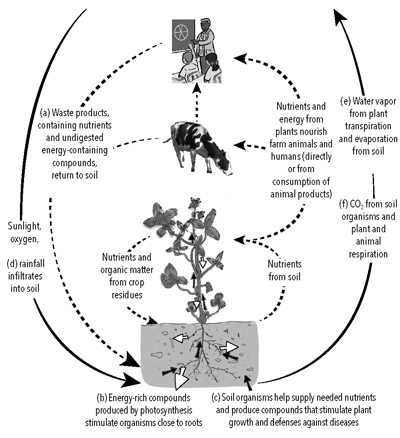
Imported Chinese laborers (“coolies”) worked on the guano islands, extracting this valuable fertilizer for export to the global North. Undernourished, physically beaten, choking on dust, they labored as beasts of burden under conditions, as noted by Marx, “worse than slavery.”[10] Today huge quantities of nitrogen fertilizer are produced by the Haber-Bosch process, while large amounts of potash and phosphorus minerals are mined and treated. The use of increasing amounts of energy (especially for nitrogen production) by the Haber-Bosch process, as well as by mining and processing of phosphorus, causes great ecological disturbance and pollution.
The metabolic rift of people removed from land that produces their food continues unabated with the transfer of large populations from rural areas to cities. This phenomenon that began in earnest with the British land foreclosures that forced peasants off the land between the fifteenth and nineteenth centuries continues today with modern foreclosures, as farmers in Latin America, Africa, and Asia are forced off the land and migrate to cities that hold few jobs for them. Most enter the “informal economy” and struggle for existence.[11]
In the second half of the twentieth century, another rupture of nutrient cycling occurred as large, integrated capitalist firms concentrated beef cattle feedlots, poultry production, and hog operations near slaughtering facilities. This overwhelmed the supplies of locally grown feed sources, forcing the importation of feed, mainly corn and soy, from hundreds, even thousands, of miles away. This had two effects: the depletion of nutrients on grain farms (forcing them to purchase large amounts of commercial fertilizers) and the accumulation of mountains of manure on animal farms (causing water pollution due to the excess level of nutrients).
A further accentuating factor was caused by a change in crop rotations. Perennial forage legumes and grass-legume mixtures were once a central part of farm rotations. The multiyear hay and pasture crops produced numerous positive effects. One of these was that the nitrogen remaining from the legumes could be supplied to following crops of grains such as corn or wheat. Tractors replaced animal traction, beef cows were increasingly raised in large feedlots, and dairy cow numbers per farm grew. Many former dairy or mixed crop-livestock farms were converted to mechanized crop farms, often raising only grains and soybeans. These “cash grain” farms had no use for rotations containing perennial legumes to feed as hay or to pasture animals—because they had no farm animals. Nor did their neighbors. Thus, many farms stopped producing their own nitrogen (from legumes) for grain crops and were thereby compelled to purchase large amounts of nitrogen fertilizer—produced with massive amounts of energy, usually natural gas. (Although soy, an annual crop, is a legume, it leaves little nitrogen available for a following grain crop.)
Figure 2. Interferences in soil-plant-animal-atmosphere metabolic interactions (click image to enlarge).
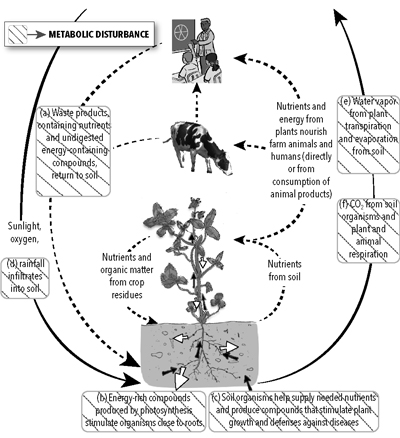
Rifts in the circulation of organic matter and the carbon cycle (see [a] and [f] in Figures 2 and 3)
When discussing soil organic matter, keep in mind that, as with all organic compounds, it is composed of carbon-rich substances. In fact, there is about three times more carbon stored in soils in the form of soil organic matter than is in the atmosphere. Agricultural practices affect how much carbon is stored in soils as organic matter and how much is released back into the atmosphere as carbon dioxide. When forests or grasslands are converted to agricultural production, there is usually a great loss of organic matter from the soil, as plowing and other disturbances accelerate the use of stored organic matter by organisms. This accelerated microbial metabolism leads to massive carbon dioxide emissions into the atmosphere. Once soil organic matter is very depleted (see below), the soil may stop being a net source of carbon dioxide to the atmosphere.
In addition to influencing nutrient cycling, the separation of humans and farm animals from the land that grows their food contributes to the break in the soil-plant-animal-human organic matter cycle. Decreased return of organic residue to the soil means that less food is provided to soil organisms. This occurs not only because manure and human waste are not returned to the field but also because of the more simplified agroecosystems that eliminate soil-building perennial hay crops from rotations. Although some crop residues remain in the soil in these simplified systems, organic matter declines or remains low, and organisms have less food, reducing the population and diversity of soil life.
Disruption of the hydrologic (water) cycle (see [d] and [e] in Figures 2 and 3)
Numerous practices of capitalist development—such as concentrating people in large cities, raising animals on factory farms, over-pumping water from aquifers for irrigation, clearcutting of forests, and mountaintop removal for coal mining—have caused a significant alteration in the hydrologic cycle in ways that have degraded local and regional ecosystems.
The enormous amount of water being pumped from aquifers—mainly to irrigate crops—is having a significant effect on the global hydrologic cycle. “People are drawing so much water from below [ground] that they are adding enough of it to the ocean (mainly by evaporation, then precipitation) to account for about 25 percent of the annual sea level rise across the planet.”12 In important agricultural areas such as northwestern India, northeastern China, northeast Pakistan, California’s central valley, and the Great Plains of the United States, waters are being pumped from aquifers in such excess that water tables are falling rapidly, threatening continued irrigated agriculture. With much of the water for irrigation coming from the world’s rivers, some rarely reach their normal outlets—for example, China’s Yellow River, the Colorado River in the United States and Mexico, and the Euphrates and Tigris Rivers in the Middle East. Although damage occurs to life in the estuaries, most of the water used for irrigation is transpired by plants and evaporated from soils and is then returned as rainfall.
As simplified agroecosystems develop, and less land is devoted to perennial hay/pasture crops, less organic matter is returned to soils (see “organic matter cycle above”). As organic matter decreases, soil structure deteriorates, and water infiltrates more slowly into the soil. Use of heavy equipment, especially when the soil is wet, causes compaction. Under these conditions, a significant portion of rainfall, especially from intense storms, runs off the land, causing soil erosion that takes organic matter-enriched topsoil. Plants thus become more dependent on irrigation, even in humid regions.
Figure 3. Reactive interventions and primary consequences (click image to enlarge).
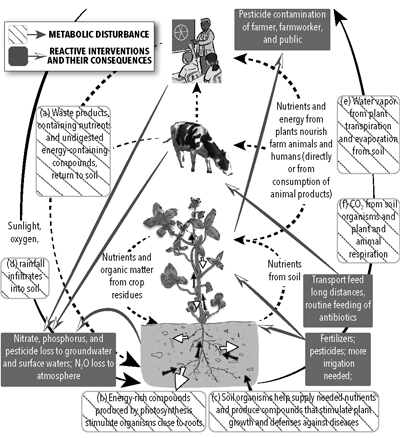
Clearfelling, the quickest way to make money from forests, leaves the soil without trees to intercept rainfall and lessen its impact. As roots die, their ability to hold onto soil decreases. Surface organic matter may also decrease, enhancing erosion. In large tropical rainforests, water vapor lost to the atmosphere by transpiration from tree leaves travels downwind and returns to the forest as precipitation. The loss of large areas of tropical rainforest greatly reduces this continual cycling of water—with more running off into streams and rivers.
As cities and suburbs and shopping centers grow, and streets are paved, there is less exposed soil for water infiltration. This results in larger runoff volumes during rains, less recharge of local aquifers, and less streamflow during periods of low rainfall.
Rifts in the interactions among organisms (see [b] and [c] in Figures 2 and 3)
Simplified agroecosystems (few crops [with homogenous genetics], short rotations, eliminating hedge and tree rows) in place of forests and grasslands, and removing animals from crop farms cause decreased plant/animal diversity aboveground and diversity of organisms belowground. There are fewer habitats for wildlife and for beneficial organisms in and around fields. Within the soil, the decreased quantities and variety of organic matter return leads to decreased soil organic matter levels and decreased diversity and organism activity. As plant, soil, and animal biodiversity decreases, outbreaks of insect pest and diseases become more common (less self-regulation), inducing the use of increasing amounts of pesticides. (An interesting experiment in China has shown that providing genetic diversity by growing different varieties of rice in alternating strips can help decrease disease prevalence.[13])
Interventions to try to bridge metabolic rifts—or transfer the problems elsewhere
Faced with the problems associated with severely degraded metabolic relationships, the main responses have been as follows (see also Figure 3): (1) transport food and feed large distances to cities and to where farm animals are concentrated, routine use of antibiotics in animal feed; (2) apply large amounts of fertilizers on crop farms to deal with the rift in the nutrient and organic matter cycles; (3) use pesticides to overcome insect, weed, disease, and nematode pests that develop with simplified systems (monocultures, poor rotations, and depleted soil organic matter); (4) use extra irrigation water to deal with decreased ability of soil to allow rainfall to infiltrate and to store water for plants; (5) transport water from water surplus regions long distances to where aquifer and river water is depleted.
The interventions then have the unintended effect of water pollution by nutrients and pesticides, and contamination of farmer, farm worker, and food with pesticides and development of antibiotic-resistant bacteria. More and more interventions are needed to obtain reasonable crop yields from soils depleted of organic matter, with low amounts of biological diversity, and greatly simplified aboveground agroecosystems.
The complexity of ecological disruptions
Marx emphasized the loss of “the lasting fertility of the soil” as a result of the net export of nutrients, as people moved (or were forced to move) to cities during the early phase of capitalist development. This created what he called an “irreparable rift in the interdependent process of social metabolism, a metabolism prescribed by the natural laws of life itself.”[14] But many of the large-scale agricultural systems are inefficient at cycling nutrients for other reasons, as well. Huge amounts of nitrogen fertilizer are lost in growing corn on most farms, as nitrate leaches into ground water, which then contaminates streams and rivers. (Significant amounts of phosphorus also tend to be lost from these systems, along with sediments in surface runoff and erosion.) Excess nitrate from the corn grown in the U.S. Midwest is believed to be the primary cause of the low-oxygen (sometimes called “dead”) zone at the mouth of the Mississippi River. There are hundreds of these low-oxygen zones around the world, the largest of which occurs in Europe’s Baltic Sea. A smaller, though significant, amount of soil nitrogen is converted to nitrous oxide and diffuses into the atmosphere where it is a potent greenhouse gas, and also destroys ozone in the stratosphere.
The need to use so much fertilizer results from losses of nutrients in runoff, the leaching and volatilization in inefficiently designed systems, and separation of people and animals from the land that produces their food. One consequence of continuous loss of nutrients from soil is the need to import fertilizers on crop farms, with severe environmental cost in terms of mining disruption and energy use. Production of nitrogen fertilizer is especially energy intensive. Approximately 1,200 m3 of natural gas is needed to produce one metric ton of anhydrous ammonia fertilizer (equivalent to about 1,700 kg of N).[15] Approximately one third of the energy used to grow a crop of corn in the U.S. Midwest is used to produce, ship, and apply nitrogen fertilizer. And every ton of phosphorus as fertilizer, after acid has been added to the rock to make it more soluble, results in five tons of waste, which contains radioactive substances and leaches highly acidic water. In addition, the world may be close to “peak phosphorus” extraction, after which phosphorus will be more expensive and difficult to obtain. The lack, or prohibitively high cost, of phosphorus fertilizers may turn out to be one of the largest disruptions in the world’s agriculture.
As discussed above, the changes in agricultural practices accompanying agricultural intensification go far beyond the issue of the depletion of soil nutrients and suggest ramifications for other metabolic connections.
Another critical issue is the abysmal treatment of labor in capitalist agricultural systems. Migrant workers, especially those from other countries, have few rights and are frequently treated as virtual slaves. Farm laborers and their families are also commonly heavily contaminated with pesticides. Conditions of labor on large agricultural plantations, mainly in Africa, Latin America, and Asia, are usually the most exploitative. Workers are paid very low wages, violence or immediate firing is used to stop unionization, and pesticides (many banned in the rich countries) are used freely, with severe health effects.
Groundwater may be contaminated with pesticides, as well. For example, Costa Rica’s coordinator for the Pesticide Action Network told a news reporter that the pineapple plantations “use organophosphates, organochlorines, hormone disruptors, chemicals that are known to cause cancer, chemicals that are reproductive toxins.”[16] And it is not just the workers and local water that are contaminated—so is the produce that people eat. Some 94 percent of the pineapples imported to Britain from Costa Rica contained “residues of the fungicide triadimefon, a reproductive toxin and suspected hormone disruptor.”[17]
The wider metabolic rifts engendered by industrial production/consumption
We have discussed almost exclusively natural systems and the causes and consequences of disturbed metabolic relationships that have occurred with the introduction and growth of capitalist agriculture. A full discussion of degraded metabolic relationships and other problems associated with the industrial/service economy in general is beyond the scope of this paper. However, we must at least note that industrial mining and refining of raw materials and production of commodities, with profit as the sole aim, result in significant environmental problems, such as: (1) numerous toxic chemicals are used (polluting human bodies and the rest of the ecosystem); (2) carbon dioxide and other pollutants are emitted by power plants and oil refineries; (3) nonrenewable resources are depleted and renewable resources are undermined and even depleted; (4) the land itself is destroyed in such ventures as “mountaintop removal” in the mining of coal; (5) the massive production of unneeded commodities for a small minority of the world’s population (and persuading them to buy them), while not meeting the basic needs for a larger portion of humanity.
The characteristics of capitalist economies derive from the motive force—the accumulation of capital without end.[18] As Richard Levins has explained, “Agriculture is not about producing food but about profit. Food is a side effect.”[19] The same could be said about the production of almost anything, or the provision of any service in a capitalist system. Capitalism, in its pursuit of accumulation of capital as means to further accumulation, “ad infinitum,” engages in extreme forms of both the division of labor and the division of nature. The result is to simplify and degrade them both, creating metabolic disturbances and rifts between human beings and nature and rupturing the entire social metabolism.
Table 1. Consequences of degradation or rift in metabolic relationships
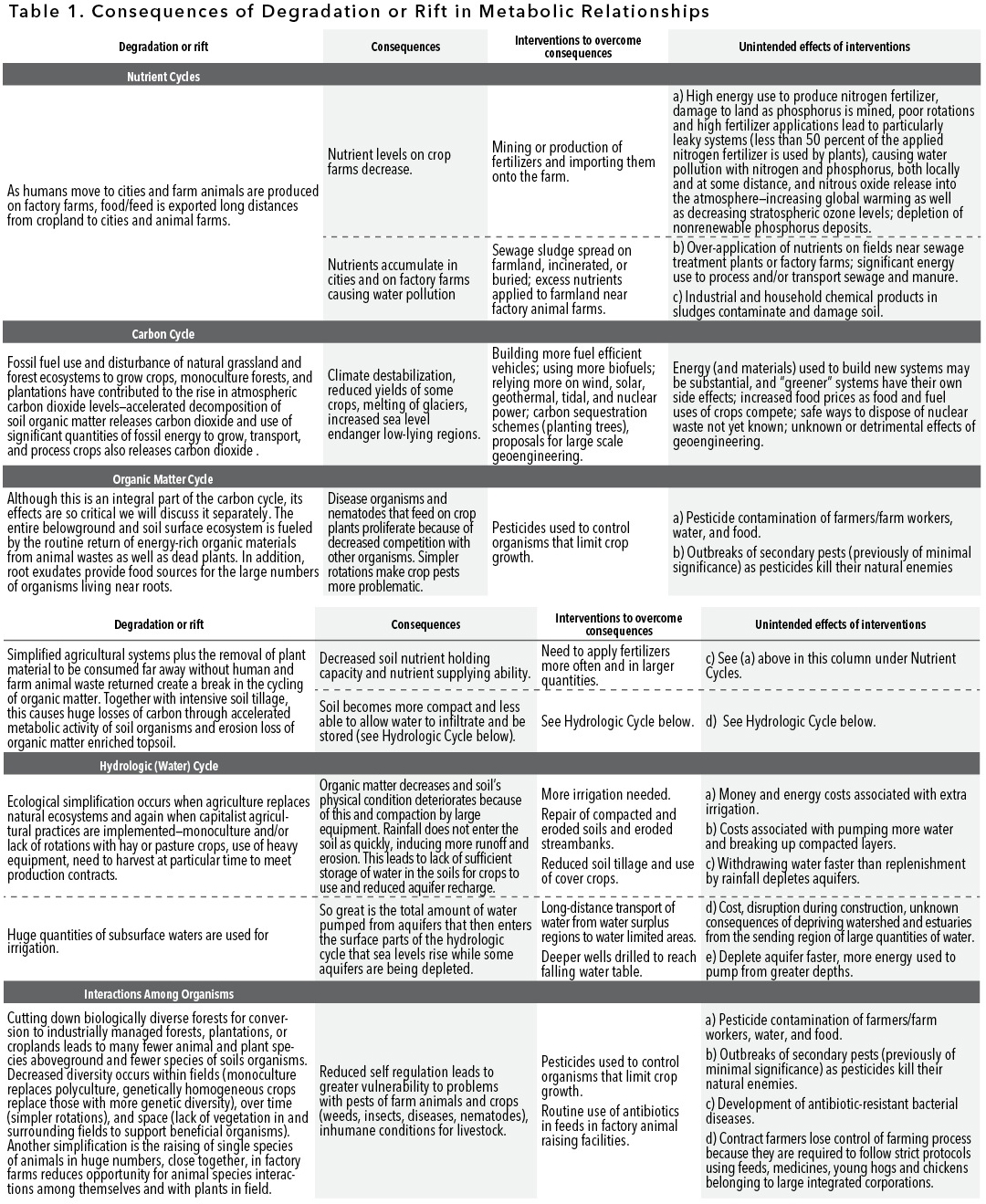
III. Creating an ecological civilization
Capitalism is incompatible with a truly ecological civilization because it is a system that must continually expand, promoting consumption beyond human needs, while ignoring the limits of nonrenewable resources (the tap) and the earth’s waste assimilation capacity (the sink). As a system of possessive individualism it necessarily promotes greed, individualism, competitiveness, selfishness, and an Après moi le déluge philosophy.[20] Engels suggested that “real human freedom” can be achieved only in a society that exists “in harmony with the laws of nature.”[21]
Although it is impossible to know what future civilizations will be like, we can at least outline characteristics of a just and ecologically based society. As a system changes, it is the history of the country and the process of the struggle that bring about a new reality. However, in order to be ecologically sound, a civilization must develop a new culture and ideology based on fundamental principles such as substantive equality. It must (1) provide a decent human existence for everyone: food, clean water, sanitation, health care, housing, clothing, education, and cultural and recreational possibilities; (2) eliminate the domination or control of humans by others; (3) develop worker and community control of factories, farms, and other workplaces; (4) promote easy recall of elected personnel; and (5) re-create the unity between humans and natural systems in all aspects of life, including agriculture, industry, transportation, and living conditions.
An ecological society is one that will need to be the opposite of capitalism in essentially all aspects. It would: (1) stop growing when basic human needs are satisfied; (2) not entice people to consume more and more; (3) protect natural life support systems and respect the limits to natural resources, taking into account needs of future generations; (4)make decisions based on long-term societal/ecological needs, while not neglecting short-term needs of people; (5) run as much as possible on current (including recent past) energy instead of fossil fuels; (6) foster the human characteristics and a culture of cooperation, sharing, reciprocity, and responsibility to neighbors and community; (7) make possible the full development of human potential; and (8) promote truly democratic political and economic decision making for local, regional, and multiregional needs.
When considering the characteristics of strong ecosystems to explore those of strong economic/social systems, there will be overlap—some vital traits clearly belong to more than one “characteristic” or “pillar.” Nevertheless, the analytical separation of these traits/characteristics helps provide a basis for organizing our thoughts on the issues involved. An ecological civilization will depend on creating appropriate human social-ecological metabolism, so that society can perpetually meet human and environmental needs. The discussion below is not meant either to be a complete outline or a blueprint, but rather to stipulate some of the crucial characteristics of an ecological civilization.
Self-regulation
Decisions are made—to as great an extent as possible—at the level where the effects will be most felt. Self-regulation in this sense is democratic self-governing and needs to occur at the workplace, community, multi-community, regional, and multiregional levels, so that major political and economic decisions are in the hands of an empowered populace. A system of economic and political democracy provides better identification of, and solutions to, problems. This system will require a public that is well educated, informed about alternative possibilities, interested, and encouraged to participate in making decisions. Although local inhabitants know their area and needs best, people must have the knowledge and analytical skills to make informed decisions. An embryonic example of this is the system of community councils in Venezuela in which one hundred to four hundred families participate in making decisions regarding investment needs in their communities and are provided resources to implement these decisions.
The labor and production process governing the human-social metabolism with nature is collectively organized and controlled. Farmers and workers in factories and offices—together with their local communities—control their workplace. People will be encouraged to participate in leadership, while a system also needs to be established for regular review of persons in authority at all levels of society—and easy removal, if necessary. All decisions will take into account the meeting of basic human needs and the allowance for the full development of human potential, while maintaining or recreating the healthy local/regional/global environment essential for healthy humans and the rest of life.
Diversity
It comes in many forms—diversity of opinions and talents (because people have different backgrounds and experiences). It can also apply to the details about how communities and regions are organized—as long as all are dedicated to similar goals. Diversity provides significant strengths and security, and adds to the community socially and culturally, as well as economically. Better decisions are made by taking into account the thoughts of people looking at the same issues through different lenses. Diversity of opportunities for education, recreation, development of particular interests strengthens the community and society. Biological diversity is encouraged through more complex agriculture cropping systems that emphasize building healthy soils, and through better integration of less disturbed (“natural”) areas within agricultural and urban landscapes.
Efficient natural cycles through closely linked metabolic relationships
The concept of capitalist efficiency—simplification of production processes, producing with the least amount of (and most unskilled) labor possible, lowering labor and other costs to reap maximum profits, using less gasoline to travel more miles in your car—will be replaced by ecological concepts of metabolic efficiency in the cycling of materials and energy that permit the sustainability of the civilization. These rely on synergies that develop among people living in cooperation with each other (instead of in competition and individualist isolation) and with nature (instead of attempting to overwhelm and dominate it). Social metabolism, or human interactions with each other, is analogous to the metabolic interactions among nonhuman organisms (microorganisms, plants, insects, other animals) in natural systems. Working fewer hours—if everyone participates to produce life’s needs—means that people will have more time to spend with family and community. And stronger communities are developed when individualism, consumerism, and competition are replaced with cooperative ties among people, as the communities develop procedures and systems for meeting the basic physical, cultural, and recreational needs for everyone.
Principles of ecological design will be used for agriculture, construction, manufacturing, and assisting recovery of damaged ecosystems. Living in cooperation with nature leads to tightly coupled nutrient, energy, and water flows, and to maintaining the functioning of natural cycles and flows. A regenerative agriculture based on ecological principles is an agriculture that works with nature, instead of against it, to create and maintain healthy and diverse habitat in and around fields and in productive soils.[22] Raising animals on farms where their feed is produced allows for efficient nutrient cycling and synergies of better crop rotations, while reducing dependence on processed mineral deposits (for phosphorus and potash) and exorbitant use of energy to produce nitrogen fertilizers. This also provides a purpose for growing perennial forage legumes and grasses more widely as feed for ruminants. If no industrial waste or other contaminants are allowed to degrade the usefulness of human sewage, we can rely on the efficient cycling of nutrients from human waste back to the land that grows our food.
People will, to as great an extent as possible, live near where they work, use public mass transit, and eat food produced in reasonable proximity to their homes. People will work fewer hours, because, with all unnecessary jobs (for example in advertizing and other parts of the sales effort, and much of finance, real estate, and insurance) eliminated, it will not take that much work to produce the basic needs of society. But everyone who can work will have a job.
An ecological civilization cannot be based on private automobiles as the main, or even a significant, transportation system. No matter how fuel efficient cars and trucks become, the use of buses and trains for the main regional transportation systems will be more energy efficient. A less car-dependent society will use up fewer materials, cause less disturbance, and use less land for road construction and all the businesses connected to an automobile culture (such as McDonald’s drive through restaurants). Luxury commodities will not be produced, and products will not come in the elaborate packaging now used, which overwhelms the earth’s landfills. People will live in homes designed to be attractive and comfortable but also to be energy efficient and to take advantage of natural heating/cooling possibilities.
Industrial production of human needs will necessarily entail some disturbance of natural systems, just as agriculture does. But care must be taken to minimize negative effects of such practices, along with mining. Production systems will be based on “cradle-to-cradle” concepts, where easy reuse of materials or structures is built into the original product.[23] Extreme forms of both the division of labor and the division of nature that create rifts within life itself will be avoided.
Self-sufficiency
Complete self-sufficiency is neither possible nor desirable for all regions and all communities. However, self-sufficiency with regard to many needs, such as food, water, housing, and energy should be a goal toward which communities and regions strive. In this way, most of the knowledge and skills needed for providing the basics reside within the local community or group of neighboring communities. Self-sufficiency may include using relatively small-scale local forms of renewable energy such as solar, wind, geothermal, or hydro instead of relying on energy generated at large facilities far away (“green” or not) and depending on long-distance transmission. Community-based food systems (production and distribution) based on an agriculture that relies on ecosystem strengths and close nutrient cycling, will run as much as possible on current energy.
Again, this does not mean that all communities and regions should be completely self-sufficient (for example, it makes no sense for all communities to produce their own buses or refrigerators). Rather, they should strive toward that goal—especially for the basic foods. Redundancy in peoples’ skills and in production facilities enhances self-sufficiency.
Resiliency through self-renewal
The degree of resiliency and self-renewal depends on how well all the traits discussed above have been developed and incorporated into society. Community and regional social structures and economies that can better withstand adverse events and recover quickly will be more sustainable. The characteristics, or pillars, discussed above—self-regulation, self-sufficiency, diversity, and efficiency through closely linked metabolic relationships—all contribute to creating a resilient society. Community and regional structures and economies based on these characteristics should be able to withstand adverse events and recover more quickly through a process of self-renewal. Global cultural interchange and cooperation (made more viable because it will be between mutually self-determining communities that are not in competition with each other) also enhance resiliency.
It is inconceivable that capitalism itself will lead directly to an ecological civilization that provides the basic needs for all people. However, building an ecological civilization that is socially just will not automatically happen in post-capitalist societies. It will occur only through the concerted action and constant vigilance of an engaged population.
[January 2011 edition of Monthly Review.]
is professor emeritus of plant and soil science at the University of Vermont and adjunct professor of crop and soil science at Cornell University. His most recent book is Agriculture and Food in Crisis (co-edited with Brian Tokar, Monthly Review Press 2010). This article is slightly revised from his presentation to the Marxism and Ecological Civilization Conference at Fudan University, Shanghai, November 17, 2010. This article first appeared in theNotes
- ↩ Frederick Engels, “The Part Played by Labour in the Transition from Ape to Man,” in Karl Marx and Frederick Engels, Collected Works, vol. 25 (New York: International Publishers, 1975), 460-61.
- ↩ Lindsay Turnbull and Andy Hector, “How to Get Even with Pests,” Nature. 466 (July 1, 2010): 36-37.
- ↩ A more detailed description of plant defense mechanisms can be found in pages 77 to 81 in Fred Magdoff and Harold van Es, Building Soils for Better Crops (3rd edition), http://sare.org/publications/bsbc/bsbc.pdf.
- ↩ Fred Magdoff, “Ecological Agriculture: Principles, Practices, and Constraints,” Renewable Agriculture and Food Systems 22, no. 2 (2007): 109–17.
- ↩ Peter Miller, “Swarm Behavior,” National Geographic, July 2007; Thomas D. Seeley, Honeybee Democracy (Princeton, NJ: Princeton University Press, 2010).
- ↩ Engels, “The Part Played by Labour,” 461.
- ↩ Ibid., 463.
- ↩ Karl Marx, Capital, vol. 1 (London: Penguin, 1976), 637.
- ↩ John Bellamy Foster and Fred Magdoff, “Liebig, Marx and the Depletion of Soil Fertility: Relevance for Today’s Agriculture,” Monthly Review 50, no. 3 (1998):32-45.
- ↩ John Bellamy Foster, Brett Clark, and Richard York, The Ecological Rift (New York: Monthly Review Press, 2010), chapter 15.
- ↩ George Packer, “The Megacity: Decoding the Legacy of Lagos,” The New Yorker, November 13, 2006; Patrick Barta and Krishna Pokharel, “Megacities Threaten to Choke India,” Wall Street Journal, May 13, 2009.
- ↩ “Groundwater Use Increasing Sea Level Rise,” a description of Bierkens, M.F.P. et al. in “A worldwide view of groundwater depletion,” Geophysical Research Letters, http://seaweb.org/news.
- ↩ Youyong Zhu, Hairu Chen, Jinghua Fan, Yunyue Wang, Yan Li, Jianbing Chen, JinXiang Fan, Shisheng Yang, Lingping Hu, Hei Leung, Tom W. Mew, Paul S. Teng, Zonghua Wang, and Christopher C. Mundt, “Genetic Diversity and Disease Control in Rice,” Nature 406 (2000): 718-22.
- ↩ Karl Marx, Capital, vol. 3 (London: Penguin, 1981), 949.
- ↩ Clark Gellings and Kelly E. Parmenter, “Energy Efficiency in Fertilizer Production and Use,” Encyclopedia: Efficient Use and Conservation of Energy, vol. 2, http://eolss.net/ebooks.
- ↩ Felicity Lawrence, “Bitter Fruit: The Truth about Supermarket Pineapple,” The Guardian [UK], October 2, 2010.
- ↩ Ibid.
- ↩ For a more detailed discussion, see Fred Magdoff and John Bellamy Foster, “What Every Environmentalist Needs to Know about Capitalism,” Monthly Review 61, no. 10 (March 2010): 1-30.
- ↩ Richard Levins, “Why Programs Fail,” Monthly Review 61, no. 10 (March 2010).
- ↩ “Après moi le déluge! is the watchword of every capitalist and every capitalist nation. Capital therefore takes no account of the health and length of life of the workers unless society forces it to do so.” Karl Marx, Capital, vol. 1, 381.
- ↩ Frederick Engels, Anti-Dühring, in Marx and Engels, Collected Works, vol. 25 (New York: International Publishers, 1975), 106.
- ↩ Magdoff, “Ecological Agriculture: Principles, Practices, and Constraints.”
- ↩ William McDonough and Michael Braungart, Cradle to Cradle (New York: North Point Press. 2002).